The Ultimate Guide to RC Batteries
Published by James: February 26, 2025
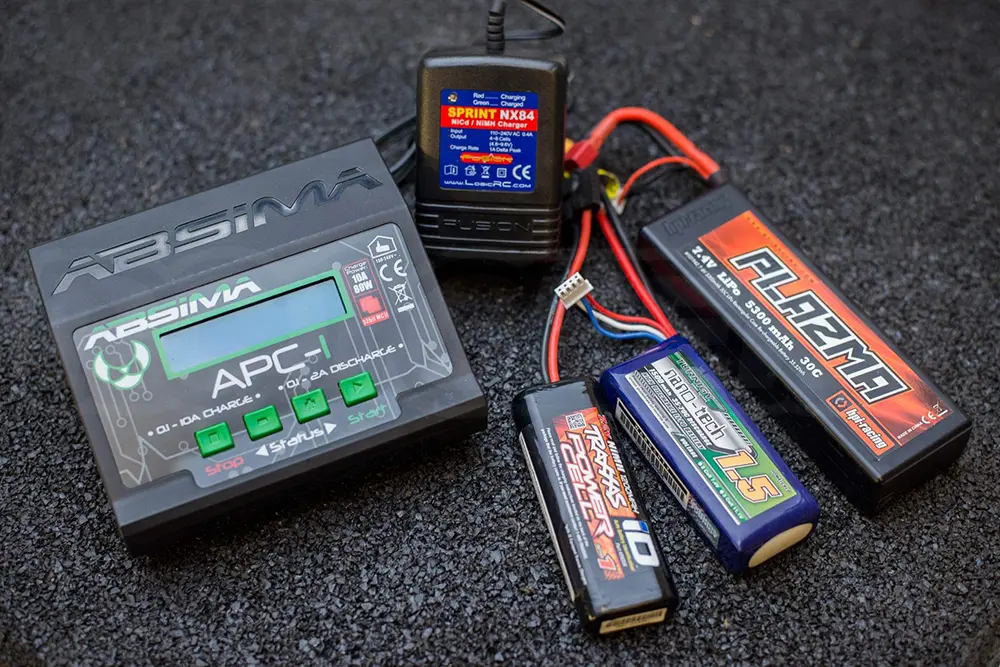
1. Overview
In RC aeronautics, batteries are more than just power sources—they’re critical performance components. Factors like energy density (how much energy can be stored per unit weight), discharge rate (the maximum current the battery can supply), weight, safety, and lifespan all play important roles. This guide covers the major types of batteries used in RC—Ni-Cad, Ni-Mh, LiPo, LiFe, and Li-Ion—and dives into their specific characteristics, charging cycles, depletion thresholds during load, storage settings, as well as a comprehensive overview of their pros, cons, and best applications.
2. Capacity, Discharge & C Rating
Definition: The capacity of a battery, given in ampere-hours (Ah) or milliampere-hours (mAh), tells you how much charge the battery can store. For example, a 2200 mAh battery can, at a 1C rate, deliver 2.2 A continuously for one hour.
The "C" Rating: The C rating is based on the battery’s capacity. A 1C discharge rate means the battery can deliver its capacity in amperes over one hour. When a battery has a higher C rating, its maximum safe continuous discharge current is calculated by multiplying the capacity by that rating. For instance, a 2200 mAh battery with a 20C rating can theoretically deliver:
2.2 Ah × 20 = 44 A
This rating is crucial in high-demand applications, such as RC vehicles or drones, where high current draws are common. Overdrawing current can lead to overheating, reduced lifespan, and even safety hazards.
Continuous vs. Burst Discharge: The continuous discharge rating is the current the battery can safely provide on a sustained basis, while the burst (or peak) rating applies for short durations.
Additionally, the battery’s capacity (e.g., 5200mAh) determines how long it can run a device at a given current draw.
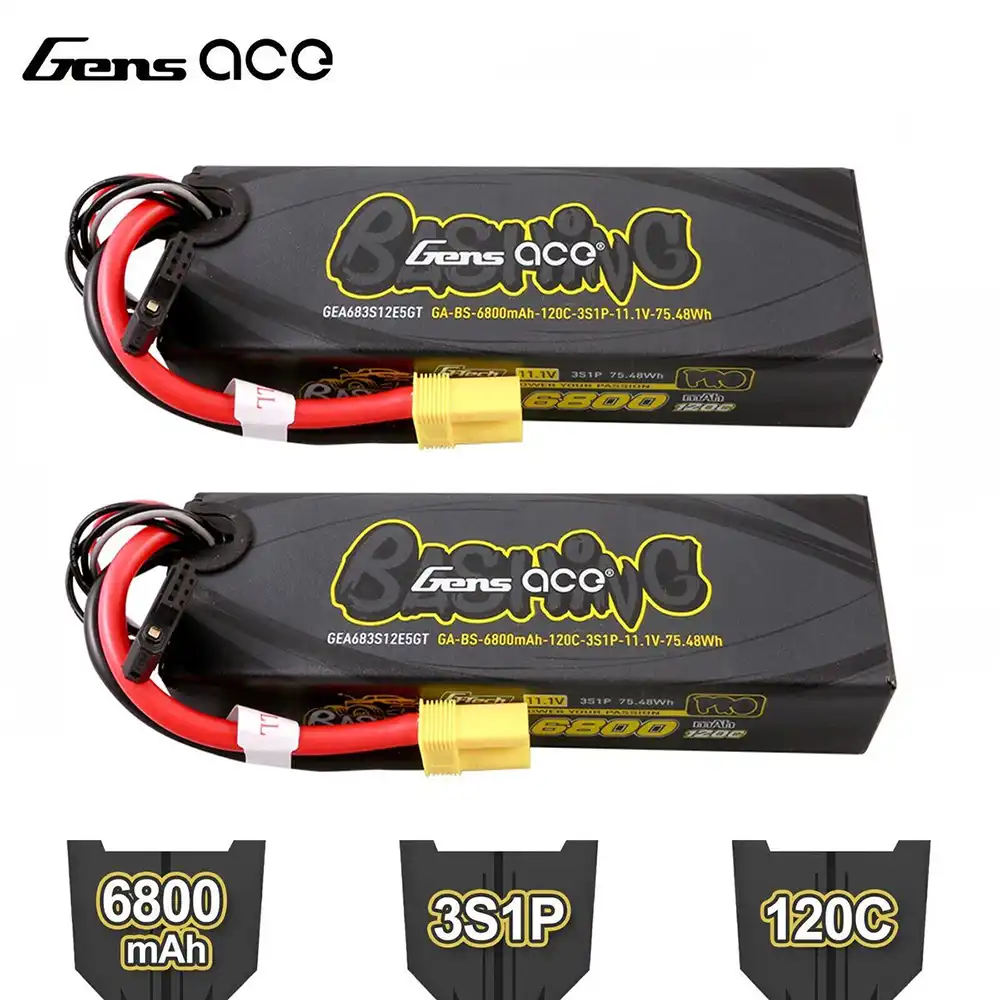
3. Cell Count and Series Configuration
The “S” Notation: When you see a designation like “4S”, the “S” stands for series. This means the battery has 4 cells connected in series.
Voltage Implications:
- LiPo Batteries: Each LiPo cell has a nominal voltage of about 3.7V (with a fully charged voltage of around 4.2V). Thus, a 4S LiPo has a nominal voltage of 4 × 3.7V = 14.8V and a maximum of about 16.8V.
- LiFe Batteries: LiFe (or LiFePO₄) cells have a lower nominal voltage (around 3.2V per cell), so a 4S LiFe battery would have a nominal voltage of roughly 12.8V.
Series vs. Parallel: Sometimes you may see a “P” notation (for example, 3S2P). Here, “P” stands for parallel, meaning two sets of 3 cells in series are connected in parallel. This increases the battery’s capacity (and thus runtime) while keeping the voltage constant.
4. Battery Chemistry Identifiers
LiPo (Lithium Polymer): Popular in high-performance RC applications due to their high energy density and ability to deliver high currents. Each cell is typically around 3.7V nominal.
LiFe or LiFePO₄ (Lithium Iron Phosphate): Known for excellent thermal stability and long cycle life, LiFe batteries have a lower nominal voltage (around 3.2V per cell) compared to LiPo.
Other Chemistries: Ni-Cad, Ni-Mh, and Li-Ion batteries are also used in RC applications. These are generally identified by their overall voltage and capacity rather than the cell configuration, and the “S” designation is most common in lithium-based batteries.
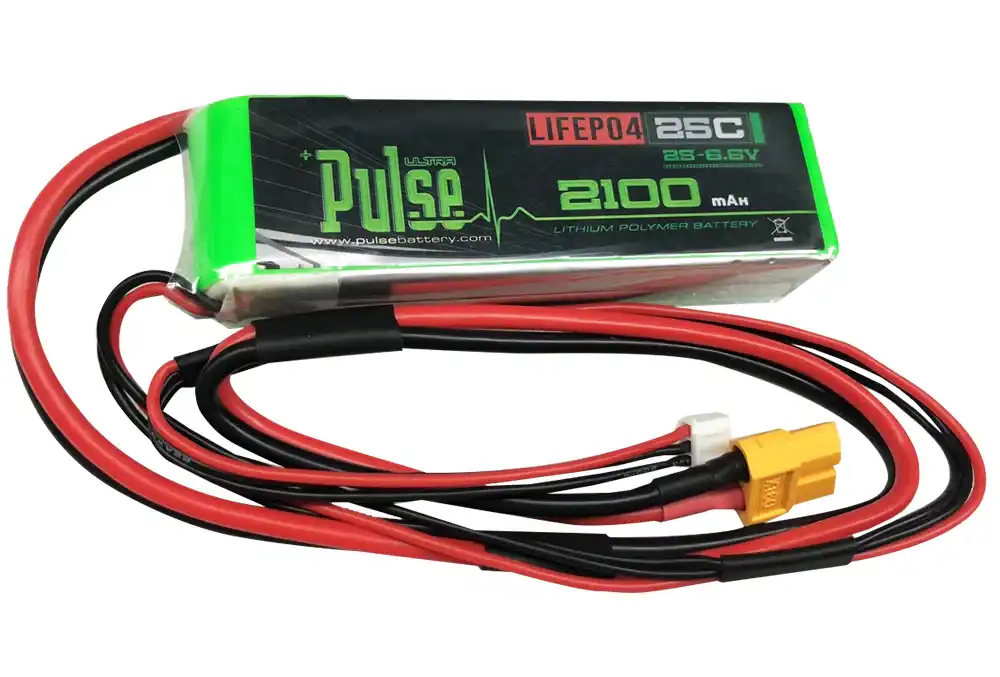
5. Putting It All Together
A complete battery description might read as follows:
- "4S 5200mAh 30C LiPo": 4 cells in series (14.8V nominal for LiPo), 5200mAh capacity, and a 30C discharge rating (allowing up to ~156A continuous), using Lithium Polymer chemistry.
- "4S 4000mAh 25C LiFe": 4 cells in series (approximately 12.8V nominal for LiFe), 4000mAh capacity, and a 25C discharge rating, using Lithium Iron Phosphate chemistry.
6. Cell Balancing Process
What is Cell Balancing?
In a battery pack composed of multiple cells, cell balancing refers to ensuring that each individual cell maintains a similar voltage and state-of-charge during both charging and discharging. Even though the cells are connected in series, slight variations (due to differences in capacity, internal resistance, or age) can lead to imbalances. The small connector with multiple thin wires on each LiPo/LiFe/Li-Ion pack is the balancing connector that connects to the charger.
Why is Cell Balancing Important?
1. Maximizing Battery Life: An imbalanced pack is limited by its weakest cell. Overcharging one cell while others lag can accelerate degradation and reduce overall lifespan.
2. Enhancing Performance: Balanced cells deliver consistent performance; if one cell discharges too quickly, the overall pack voltage can drop unevenly, affecting performance.
3. Safety: Imbalances can lead to overcharging or over-discharging individual cells, which might cause swelling, damage, or even fire. Balancing minimizes these risks.
How Does Cell Balancing Work?
There are two main methods:
• Passive Balancing: Uses resistors across each cell to dissipate excess energy as heat once a cell reaches its maximum voltage, allowing lower-voltage cells to catch up. It’s simple and cost-effective but less energy-efficient.
• Active Balancing: Transfers energy from higher-charged cells to lower-charged ones using inductors, capacitors, or specialized circuitry. This method is more energy-efficient and faster but requires more complex and expensive circuitry.
When Does Cell Balancing Occur?
• During Charging: Modern chargers often monitor individual cell voltages during the final charging phase, diverting excess energy from cells that reach their voltage threshold early.
• During Discharging: Some battery management systems (BMS) monitor cell voltages to prevent any cell from dropping below safe limits.
• Integrated in BMS: High-quality battery packs typically include a BMS that continuously monitors and balances cells throughout the battery’s lifecycle.
Summary of Cell Balancing:
Effective cell balancing is essential to maximize battery capacity, extend lifespan, and ensure safe operation. Whether through passive or active methods, maintaining uniformity across all cells helps safeguard your RC battery pack against premature failure and performance issues.
7. Ni-Cad Batteries
Nickel-Cadmium (Ni-Cad) batteries have long been a staple in RC applications due to their ruggedness and high discharge capabilities.
Key Characteristics
- Chemistry & Structure: Ni-Cad batteries use nickel oxide hydroxide and cadmium. They are robust and capable of delivering high current—ideal for high-load applications.
-
Charge Cycle & Settings:
- Charging: Typically charged using a constant current method at 0.1C for 14–16 hours.
- Depletion Threshold: Do not discharge below 1.0V per cell during flight.
- Storage: Best stored fully charged or with a slight overcharge to minimize the memory effect.
- Usage Tips: Regular full charge/discharge cycles help minimize the memory effect. A controlled trickle charge can be used during storage.
8. Ni-Mh Batteries
Nickel-Metal Hydride (Ni-Mh) batteries improve on Ni-Cads by offering higher energy density and reduced memory effects.
Key Characteristics
- Chemistry & Structure: Utilizes a hydrogen-absorbing alloy, providing higher capacity and improved energy density.
-
Charge Cycle & Settings:
- Charging: Use a smart charger with delta-peak detection at a rate of 0.1C to 0.3C (faster charging is possible with proper temperature monitoring).
- Depletion Threshold: Avoid discharging below approximately 1.0–1.1V per cell in flight.
- Storage: Store at about 40–50% charge to prolong battery life and reduce self-discharge.
- Usage Tips: Ideal for moderate load applications; avoid rapid charging without proper monitoring.
9. LiPo Batteries
Lithium Polymer (LiPo) batteries have revolutionized RC power systems with exceptional energy density and high discharge rates.
Key Characteristics
- Chemistry & Structure: Uses a polymer electrolyte that allows for flexible packaging and lightweight designs.
-
Charge Cycle & Settings:
- Charging: Require a specialized balance charger using a constant-current/constant-voltage (CC/CV) profile; typical charging rates are between 1C and 2C.
- Depletion Threshold: Considered depleted when cell voltage drops to around 3.4–3.5V; pilots often set a cutoff to protect the cells.
- Storage: For long-term storage, charge to a “storage voltage” of about 3.8V per cell.
- Usage Tips: Always monitor temperature and voltage during use; proper balancing is essential, and never store fully charged or completely empty.
-
Pros:
- High energy density for excellent power-to-weight ratio.
- High discharge rates ideal for rapid accelerations.
- Flexible form factor for custom builds.
-
Cons:
- Requires careful handling and monitoring.
- Shorter cycle life if not properly maintained.
- Strict maintenance requirements to avoid safety hazards.
10. LiFe Batteries
Lithium Iron Phosphate (LiFe) batteries, also known as LiFePO₄, are renowned for their enhanced safety and long cycle life.
Key Characteristics
- Chemistry & Structure: Utilize iron phosphate as the cathode, offering excellent thermal and chemical stability.
-
Charge Cycle & Settings:
- Charging: Charged using a similar CC/CV method as LiPo batteries, but with a lower maximum voltage (around 3.65V per cell) and typical rates between 0.5C and 1C.
- Depletion Threshold: Avoid dropping below 2.5–2.8V per cell during use.
- Storage: Store at about 3.3–3.4V per cell.
- Usage Tips: Ideal for applications where safety and longevity are prioritized over weight.
-
Pros:
- Enhanced safety and stability.
- Longer cycle life.
- Stable voltage output throughout discharge.
-
Cons:
- Lower energy density and heavier compared to LiPo.
- Reduced peak discharge capability.
- Lower nominal voltage may require more cells for equivalent voltage.
11. Li-Ion Batteries
Lithium-Ion (Li-Ion) batteries are widely used in consumer electronics and find niche use in RC models due to their high energy density and stable performance.
Key Characteristics
- Chemistry & Structure: Offer a high energy-to-weight ratio and consistent performance, though they are more sensitive to high discharge rates.
-
Charge Cycle & Settings:
- Charging: Charged using a CC/CV method at typical rates of 0.5C to 1C, with strict avoidance of overcharging.
- Depletion Threshold: Typically cut off at around 3.0V per cell to preserve battery health.
- Storage: Best stored at 40–60% capacity (approximately 3.7–3.8V per cell).
- Usage Tips: Suitable for RC models with moderate power demands; less optimized for high-discharge applications without proper management.
-
Pros:
- Very high energy density.
- Good cycle life with proper management.
- Stable voltage throughout discharge.
-
Cons:
- Lower discharge currents compared to high-performance batteries.
- Safety concerns if overcharged or damaged.
- Less optimized for the high current bursts of RC applications.
Li-Ion Cell Types
The “18650” designation is a standardized name for a cylindrical Li-Ion cell that is 18 mm in diameter and 65 mm in length. These cells are popular in a wide range of applications—from laptop batteries to electric vehicles— and are widely used in RC because they offer a good balance of energy density and reliability. Other common Li-Ion cell types include:
- 14500: Approximately 14 mm in diameter and 50 mm in length, often used in smaller devices.
- 21700: About 21 mm in diameter and 70 mm in length, providing higher capacity and increasingly used in high-performance applications.
- 26650: Roughly 26 mm in diameter and 65 mm in length, typically found in high-drain applications like power tools and high-powered flashlights.
Additionally, Li-Ion batteries are also available in prismatic and pouch formats. These formats do not follow the same simple dimension-based naming convention and are usually identified by their capacity in watt-hours or specific model numbers.
12. Overview: Pros, Cons and Best Applications
Ni-Cad Batteries
- Pros: Robust, excellent high-current performance, inexpensive and widely available.
- Cons: Memory effect if not properly cycled; lower energy density.
- Best Applications: High-load models (e.g., RC cars and helicopters) and extreme temperature environments.
Ni-Mh Batteries
- Pros: Higher energy density than Ni-Cads, reduced memory effect, and more eco-friendly.
- Cons: Higher self-discharge and sensitive to overcharging.
- Best Applications: Moderate load applications (e.g., beginner RC planes and cars) where performance and cost are balanced.
LiPo Batteries
- Pros: Exceptional energy density, high discharge rates, lightweight and compact.
- Cons: Requires careful handling and monitoring; shorter cycle life if not maintained properly.
- Best Applications: High-performance RC drones, planes, and racing models where weight and power are critical.
LiFe Batteries
- Pros: Superior safety and thermal stability, long cycle life, and consistent performance.
- Cons: Lower energy density and heavier than LiPo.
- Best Applications: Utility RC models and heavy-duty applications prioritizing safety and longevity.
Li-Ion Batteries
- Pros: High energy density, stable performance, and common in consumer electronics.
- Cons: Less suited for high discharge scenarios; sensitive to over-discharge.
- Best Applications: RC models with moderate power demands and long run times where peak discharge isn’t critical.
13. To Summarize
Choosing the right battery for your RC model involves balancing performance, safety, and cost. Whether you opt for the rugged reliability of Ni-Cads, the improved energy density of Ni-Mh, the high-performance output of LiPo, the stability of LiFe, or the energy density of Li-Ion, understanding each battery’s charge cycle, optimal discharge limits, and storage requirements is key. By breaking down each part of the label—from cell count and voltage to capacity, discharge ratings, and battery chemistry—you can make informed decisions and maximize your RC battery’s performance and longevity.
Happy flying, driving, or boating with your RC models!
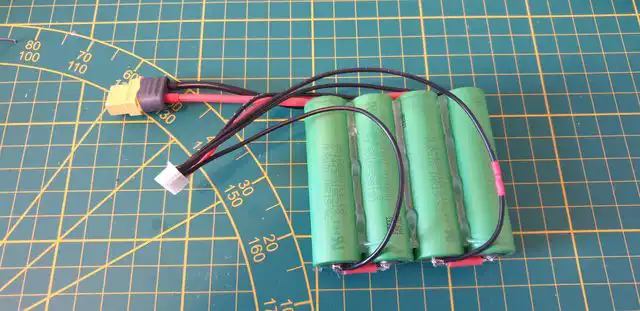